- Biodesign Academy
- Posts
- AI-Driven Protein Engineering: What it Means for Biodesign Practice
AI-Driven Protein Engineering: What it Means for Biodesign Practice
Lessons from a curious case of a tropical enzyme
There's an undeniable excitement around artificial intelligence in biotechnology right now, particularly in the area of protein engineering. And as a member of the biodesign community, I find this moment rather inspiring: AI is opening doors to design and fabricate with potentially entirely new biomaterials and processes made possible by engineered proteins.
Proteins, the nanoscopic workhorses in all living organisms, help cells communicate, break down waste, and create materials like silk and leather. Their engineering—the practice of designing and creating proteins that perform specific functions—is undergoing a revolution thanks to AI. This transformation holds promise for sustainable design practices across various industries.
In the following case study involving a recently discovered protein called “mgCel6A”, I will illustrate the potential opportunities that AI brings to protein engineering for sustainable design, and also unpack what this technological shift means for biodesign practice as a whole.
A Case Study: mgCel6A and Sustainable Biodesign
Nature has evolved remarkable enzymes like mgCel6A, which was discovered and characterized from a compost heap, by researchers Marianne Jensen and colleagues in 2018.

Protein structure of mgCel6A (image from Jensen et al., 2018, https://journals.plos.org/plosone/article?id=10.1371/journal.pone.0197862)
mgCel6A is a type of protein that thrives in tropical conditions and efficiently breaks down cellulose at high temperatures. For regions with abundant plant waste—such as Brazil, India, and Indonesia with their sugarcane and palm residues—such enzymes could revolutionize bioprocessing.
Tropical regions produce massive amounts of cellulose-rich agricultural waste from crops like sugarcane, palm oil, banana, and rice. Traditionally, much of this waste is burned or discarded, causing environmental damage.
However, mgCel6A, a highly thermostable cellulase, can transform these waste materials into valuable bioproducts, making sustainable fashion, textiles, packaging, and design materials more viable.
Regional Biodesign Applications: Nature's Enzyme at Work
The enzyme mgCel6A offers transformative solutions in tropical regions, by efficiently processing tropical biomass across multiple design sectors. The textile industry in tropical regions traditionally relied on water-intensive cotton while overlooking abundant local fibers due to their coarseness.
The mgCel6A enzyme breaks down rigid cellulose structures into softer materials, functioning optimally in the high temperatures of tropical environments. This could thus enable innovations across Southeast Asia, similar to case studies showing undervalued banana fibers becoming silk-like alternatives for luxury markets, and Bangladesh converting jute into breathable performance wear competing with synthetic fabrics.
For leather alternatives, mgCel6A could also convert agricultural waste into genuine biopolymers, for instance, to form part of fiber-softening or degumming processes towards plant-based leather alternatives.
Regarding packaging, mgCel6A could convert agricultural cellulose waste into biodegradable nanocellulose films that maintain integrity in humid environments without synthetic coatings. This may lead to innovations like rice straw-based garment bags in Vietnam replacing plastic packaging, and in Malaysia, coconut fiber becoming the raw material for compostable takeaway containers.
These applications demonstrate how new enzyme-based technologies could suit tropical contexts—thriving in high temperatures while efficiently processing regional plant waste. This creates opportunities for localized biomanufacturing that reduces fossil fuel dependence by transforming agricultural byproducts into valuable design materials.
The Challenge: From Natural Discovery to Industrial Implementation
Despite mgCel6A's impressive potential, the transition from natural discovery to industrial implementation is rarely straightforward. Many designers and bioengineers encounter significant hurdles when attempting to incorporate naturally occurring enzymes into industrial processes.
Protein Production Problems in Lab Settings
New enzymes discovered in environmental samples (like mgCel6A) often don't grow well in common lab microbes. Unlike enzymes taken directly from specific organisms, these environmental enzymes frequently don't fold correctly when produced in standard lab bacteria. A biofuel company might spend significant resources trying to produce mgCel6A in E. coli, but still face ongoing production problems.
Cost Barriers in Enzyme Development
Improving enzymes traditionally requires substantial financial investment. Researchers typically must test thousands of protein variants to find better performers, using time-consuming methods like random mutation and directed evolution. This process can take years and cost millions before creating commercially useful results—a particular challenge for developing industries with limited research budgets.
Unclear Ownership Rights
The environmental origin of these enzymes creates ownership complications. Companies may be reluctant to build production systems around enzymes with uncertain intellectual property status, as this ambiguity makes commercialization difficult and might limit market protection.
The Temperature Matching Challenge
Surprisingly, an enzyme's strength can sometimes be a limitation. While mgCel6A's ability to withstand extreme heat makes it perfect for composting, many industrial processes—especially in European settings—run at more moderate temperatures (40-60°C). In these conditions, an enzyme that's too heat-stable might actually work less effectively. The ideal enzyme needs to match the specific temperature requirements of its intended use.
AI-Driven Solutions for Protein Engineering
Computational approaches to enzyme engineering offer a promising alternative to these challenges. Rather than attempting to force naturally occurring enzymes into industrial settings, AI allows biodesigners to modify industrially friendly enzymes like TfCel6A to incorporate the beneficial properties of mgCel6A. This approach provides several advantages:

Side by side protein structure comparison of three similar enzymes, mgCel6A, TfCel6A, TfCel6B (image from Jensen et al., 2018)

Amino acid sequence comparison between those from three similar enzymes, mgCel6A, TfCel6A, TfCel6B (image from Jensen et al., 2018)
Powerful platforms like AlphaFold and Cradle which can predict and optimize protein structures with remarkable accuracy, are revolutionizing this field. These tools enable virtual experimentation that was previously impossible, allowing designers to visualize and manipulate protein structures before committing to laboratory work. This approach provides several advantages:
Predictive modification: AI can identify specific amino acid changes needed to optimize TfCel6A for mgCel6A-like performance without sacrificing expression compatibility.
Efficient development: Computational design significantly reduces the time and resources required for optimization by narrowing the experimental focus to the most promising variants.
IP clarity: Modified versions of established enzymes can be patented as novel entities, providing clearer commercialization pathways.
Customized stability: AI allows fine-tuning of thermal properties to match specific application requirements rather than defaulting to maximum possible stability.
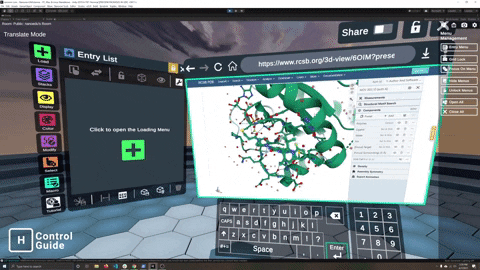
Gif by Nanome on Giphy
Lessons Learned: What Ai-Driven Protein Engineering Mean for Biodesigners
The mgCel6A case study shows several crucial insights that can fundamentally reshape how biodesigners approach their practice, offering both practical knowledge and strategic frameworks for navigating the complex intersection of biological systems and design applications. Namely:
Understanding biological constraints: Natural enzymes like mgCel6A, despite their impressive properties, can't simply be dropped into industrial processes. This reality helps biodesigners develop more realistic expectations about working with biological materials and avoid costly dead-ends in their projects.
Cost-benefit analysis framework: The economic comparison between traditional and AI-driven approaches provides biodesigners with a framework to evaluate technology investments. This is particularly valuable for independent designers or small studios with limited R&D budgets who need to make strategic decisions about technology adoption.
Temperature considerations in material processing: The insight that "more stable" isn't always better significantly impacts how designers approach enzyme selection for moderate-temperature applications like textile processing or biodegradable packaging development.
Action Points for Biodesigners
Shift from discovery to design thinking: The most fundamental change suggested is moving from simply using what nature provides to designing biological tools specifically suited to design applications. This represents a significant mindset change from adaptation to intentional creation.
Consider regional context in material development: Different regions have different biological resources and industrial capabilities. Biodesigners can use this perspective to develop more regionally appropriate material systems rather than pursuing one-size-fits-all approaches.
Engage with computational tools earlier: Rather than starting with laboratory experimentation, biodesigners could incorporate computational modeling into the earliest stages of their design process. This might mean collaborating with computational biologists or learning to use accessible AI platforms like AlphaFold or Cradle.
Focus on industrial compatibility from the start: Instead of developing materials with perfect ecological credentials that prove industrially unviable, biodesigners should consider production and scaling constraints from the beginning of the design process.
Address IP strategy proactively : Biodesigners need to consider IP strategy early in development, particularly when working with metagenomic sources.
What’s Next?
As we continue to explore the intersection of biological systems, AI, and design, our focus shifts toward equipping biodesigners with practical AI and related digital tools to overcome the challenges we've discussed.
In upcoming articles and other digital offerings, we'll aim to provide accessible guidance for biodesigners eager to incorporate AI tools into their practice. Feel free to let us know if this is something you would like to see in the near future. We could for example, provide you with:
Step-by-step tutorials designed specifically for non-specialists who want to engage with computational biology tools without extensive technical training.
Guidelines for creating effective collaborations between designers and computational biologists, fostering partnerships that combine creative vision with scientific rigor.
We look forward to hearing from you, and these resources would help towards democratized access to advanced biological design tools, opening new possibilities for sustainable innovation across industries.
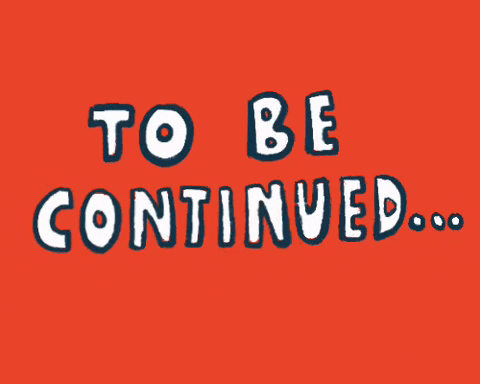
Gif by abitan on Giphy